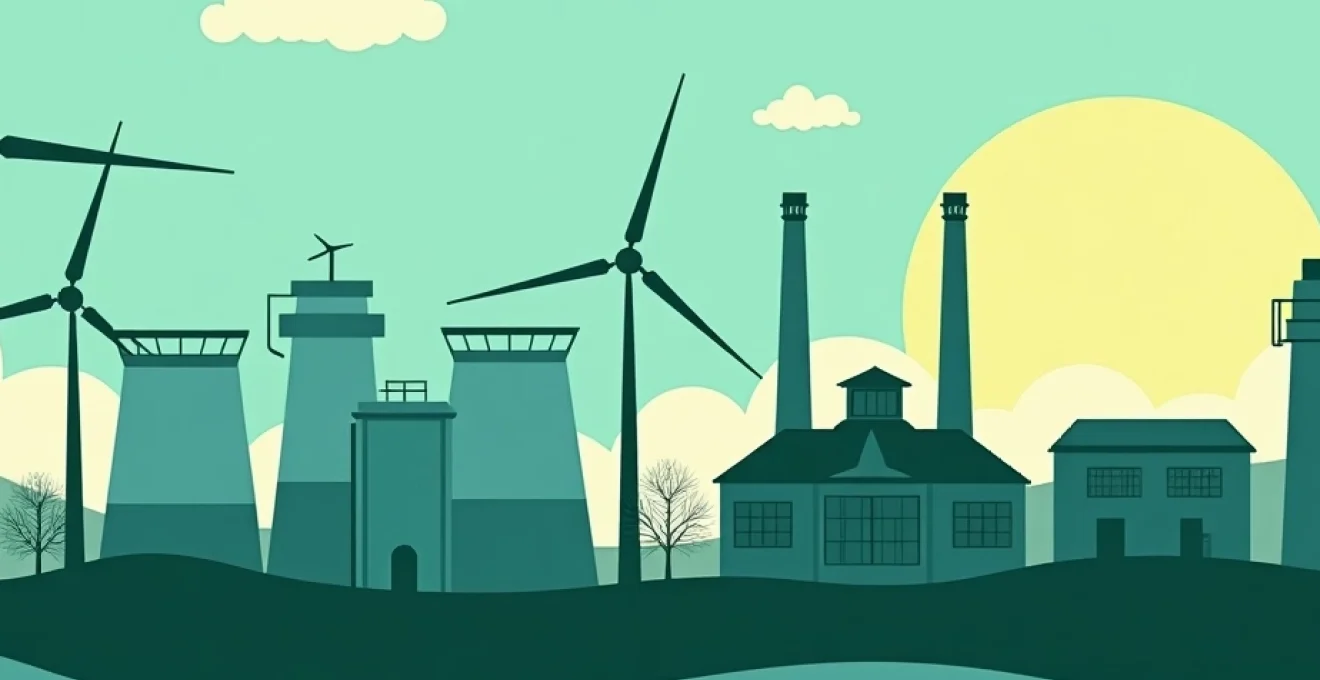
Green technologies are rapidly reshaping our world, offering innovative solutions to some of the most pressing environmental challenges we face. From revolutionizing energy production to transforming urban landscapes, these cutting-edge advancements are paving the way for a more sustainable and eco-friendly future. As we grapple with the urgent need to address climate change and reduce our carbon footprint, green technologies are emerging as powerful tools in our arsenal, promising to redefine how we live, work, and interact with our planet.
Renewable energy innovations transforming power generation
The renewable energy sector is experiencing a surge of innovation, with new technologies pushing the boundaries of what's possible in clean power generation. These advancements are not only making renewable energy more efficient but also more accessible and cost-effective, accelerating the global transition away from fossil fuels.
Advanced solar photovoltaic technologies: perovskite and multi-junction cells
Solar energy has long been at the forefront of the renewable revolution, but recent breakthroughs are taking its potential to new heights. Perovskite solar cells, a relatively new technology, are generating excitement in the scientific community due to their remarkable efficiency and low production costs. These cells can be manufactured using simple printing methods, making them a game-changer for widespread solar adoption.
Multi-junction cells, on the other hand, are pushing the limits of solar efficiency by combining multiple layers of photovoltaic materials. Each layer is designed to capture a different part of the light spectrum, resulting in overall higher energy conversion rates. Some multi-junction cells have achieved efficiencies of over 40%, far surpassing traditional silicon solar cells.
Next-generation wind turbines: offshore floating platforms and airborne wind energy systems
Wind energy is taking to the seas and skies with innovative new designs. Offshore floating wind platforms are unlocking vast energy potential in deep waters where traditional fixed-bottom turbines cannot be installed. These floating giants can be placed in areas with stronger, more consistent winds, significantly increasing energy output.
Meanwhile, airborne wind energy systems (AWES) are reimagining wind power by harnessing high-altitude winds. These systems use tethered kites or drones to capture wind energy at altitudes where winds are stronger and more constant. AWES technology promises to make wind energy viable in areas previously thought unsuitable for traditional turbines.
Emerging geothermal power: Enhanced Geothermal Systems (EGS) and closed-loop technologies
Geothermal energy is getting a boost from new technologies that expand its potential beyond traditional geothermal hotspots. Enhanced Geothermal Systems (EGS) create artificial reservoirs by fracturing hot rock formations and injecting water to create steam. This technique opens up geothermal power generation in areas previously lacking the necessary natural conditions.
Closed-loop geothermal systems are another promising innovation. These systems circulate a working fluid through sealed underground pipes, eliminating the need for water injection and reducing the risk of induced seismicity. This technology makes geothermal energy more environmentally friendly and applicable in a wider range of geological settings.
Sustainable transportation revolution: electric and hydrogen vehicles
The transportation sector is undergoing a dramatic transformation, with electric and hydrogen-powered vehicles leading the charge towards a cleaner, more sustainable future. These technologies are not only reducing emissions but also redefining the very nature of personal and public transportation.
Solid-state batteries: breakthrough in energy density and safety
Solid-state batteries represent the next leap forward in electric vehicle (EV) technology. Unlike conventional lithium-ion batteries, solid-state batteries use a solid electrolyte, which offers several advantages. They promise higher energy density, faster charging times, and improved safety due to their non-flammable nature. Major automakers and tech companies are investing heavily in this technology, with some projecting commercial availability within the next few years.
The potential impact of solid-state batteries extends beyond just increased range for EVs. Their improved energy density could lead to smaller, lighter batteries, potentially revolutionizing the design and efficiency of electric vehicles. Moreover, the enhanced safety features could alleviate concerns about battery fires, further accelerating EV adoption.
Fuel cell electric vehicles (FCEVs): Toyota Mirai and Hyundai NEXO leading the charge
While battery electric vehicles have dominated the eco-friendly car market, hydrogen fuel cell electric vehicles (FCEVs) are emerging as a compelling alternative. FCEVs generate electricity onboard by combining hydrogen with oxygen, emitting only water vapor as a byproduct. This technology offers quick refueling times and longer ranges, addressing some of the limitations of battery-powered EVs.
Toyota's Mirai and Hyundai's NEXO are at the forefront of FCEV technology, demonstrating the viability of hydrogen-powered vehicles for everyday use. These vehicles are particularly promising for long-distance travel and heavy-duty applications where battery weight and charging times can be problematic. As hydrogen infrastructure expands, FCEVs could play a crucial role in diversifying the sustainable transportation landscape.
Autonomous electric vehicles: integrating AI for optimized energy consumption
The convergence of electric vehicle technology and artificial intelligence is giving rise to a new generation of autonomous EVs. These vehicles use advanced AI algorithms to optimize energy consumption, route planning, and driving behavior. By predicting traffic patterns, adjusting speed for maximum efficiency, and coordinating with smart city infrastructure, autonomous EVs can significantly reduce energy waste and improve overall transportation efficiency.
Furthermore, the integration of AI in electric vehicles opens up new possibilities for shared mobility services. Autonomous electric taxis and shuttles could revolutionize urban transportation, reducing the need for private car ownership and optimizing vehicle utilization. This shift has the potential to dramatically decrease urban congestion and emissions while improving accessibility to transportation for all.
Smart grid technologies for efficient energy distribution
As renewable energy sources become more prevalent, the need for a more flexible and intelligent power grid has never been greater. Smart grid technologies are transforming how electricity is distributed, managed, and consumed, paving the way for a more efficient and resilient energy system.
Advanced Metering Infrastructure (AMI) and real-time pricing models
Advanced Metering Infrastructure (AMI) is revolutionizing how utilities and consumers interact with the power grid. Smart meters, a key component of AMI, provide real-time data on electricity consumption, enabling more accurate billing and giving consumers greater insight into their energy usage patterns. This technology empowers consumers to make informed decisions about their energy consumption, potentially reducing overall demand and costs.
Real-time pricing models, enabled by AMI, allow electricity rates to fluctuate based on current demand and supply conditions. This dynamic pricing encourages consumers to shift their energy usage to off-peak hours when electricity is cheaper and more abundant. For example, you might choose to run your dishwasher or charge your electric vehicle during late-night hours when rates are lower. This load-shifting helps balance grid demand and can reduce the need for expensive peaker plants that are only used during high-demand periods.
Distributed Energy Resources (DER) integration: microgrids and virtual power plants
The integration of Distributed Energy Resources (DER) is fundamentally changing the structure of our power systems. Microgrids, small-scale power grids that can operate independently or in conjunction with the main grid, are enhancing energy resilience and efficiency. These localized systems can incorporate various energy sources, including solar panels, wind turbines, and energy storage systems, providing communities with more control over their energy supply and reducing reliance on centralized power plants.
Virtual Power Plants (VPPs) take the concept of distributed energy a step further by aggregating multiple DERs into a single, coordinated network. By leveraging sophisticated software and real-time data, VPPs can optimize the output of diverse energy resources, balancing supply and demand across a wider area. This technology enables smaller energy producers, including residential solar panel owners, to participate in the energy market, potentially earning revenue by selling excess power back to the grid during peak demand periods.
Grid-scale energy storage: flow batteries and Compressed Air Energy Storage (CAES)
As the share of intermittent renewable energy sources like wind and solar increases, grid-scale energy storage solutions become crucial for maintaining a stable and reliable power supply. Flow batteries are emerging as a promising technology for long-duration energy storage. Unlike traditional batteries, flow batteries store energy in liquid electrolytes, allowing for easy scaling by simply increasing the size of the storage tanks. This makes them ideal for applications requiring several hours or even days of energy storage.
Compressed Air Energy Storage (CAES) is another innovative approach to grid-scale energy storage. This technology uses excess electricity to compress air, which is stored in underground caverns or specially designed tanks. When energy is needed, the compressed air is released, heated, and used to drive turbines that generate electricity. CAES systems can store large amounts of energy for extended periods, making them particularly useful for balancing seasonal variations in renewable energy production.
Green building technologies and sustainable urban planning
The built environment is a significant contributor to global carbon emissions, but green building technologies and sustainable urban planning strategies are offering solutions to reduce this impact. These innovations are not only making our buildings more energy-efficient but also creating healthier, more livable urban spaces.
Smart building management systems: IoT-enabled energy optimization
Smart building management systems are revolutionizing how we control and optimize energy use in commercial and residential buildings. These systems leverage Internet of Things (IoT) technology to collect real-time data from various sensors and devices throughout a building. This data is then analyzed to automatically adjust heating, cooling, lighting, and other systems for maximum efficiency.
For example, occupancy sensors can detect when a room is empty and automatically turn off lights or adjust temperature settings. Smart thermostats can learn occupants' preferences and schedules, optimizing comfort while minimizing energy waste. Some advanced systems even incorporate weather forecasts and energy price data to make predictive adjustments, further enhancing efficiency and cost savings.
Urban greening initiatives: vertical forests and biophilic design in architecture
Urban greening initiatives are transforming cityscapes, bringing nature back into our concrete jungles. Vertical forests, pioneered by projects like the Bosco Verticale in Milan, integrate thousands of trees and plants into high-rise buildings. These living facades not only absorb CO2 and produce oxygen but also provide natural insulation, reducing the building's energy needs for heating and cooling.
Biophilic design takes the concept of integrating nature into our built environment even further. This approach seeks to satisfy our innate need to connect with nature by incorporating natural elements, materials, and patterns into architectural design. Biophilic buildings often feature abundant natural light, indoor gardens, water features, and natural ventilation systems. These elements not only improve energy efficiency but also enhance occupant well-being, productivity, and overall quality of life.
Circular economy and waste-to-energy technologies
The concept of a circular economy is gaining traction as a sustainable alternative to our current "take-make-dispose" linear economic model. This approach aims to eliminate waste and maximize resource efficiency through recycling, reuse, and innovative waste-to-energy technologies.
Advanced recycling technologies: chemical recycling and molecular sorting
Chemical recycling is emerging as a game-changer in plastic waste management. Unlike traditional mechanical recycling, which is limited by contamination and degradation of plastic quality, chemical recycling breaks down plastics into their molecular components. These can then be used as raw materials for new plastics or other chemical products, effectively closing the loop on plastic waste.
Molecular sorting technologies are taking recycling to a new level of precision. These systems use advanced spectroscopic techniques to identify and sort materials based on their chemical composition. This allows for the separation of different types of plastics, metals, and other materials with unprecedented accuracy, significantly improving the quality and value of recycled materials.
Organic waste-to-energy: anaerobic digestion and biogas upgrading
Anaerobic digestion is a biological process that converts organic waste into biogas and nutrient-rich digestate. This technology is particularly effective for managing food waste, agricultural residues, and sewage sludge. The biogas produced can be used directly for heating or electricity generation, or it can be upgraded to biomethane for injection into natural gas networks or use as a vehicle fuel.
Recent advancements in biogas upgrading technologies are making it easier and more cost-effective to produce high-quality biomethane. Membrane separation and pressure swing adsorption techniques can remove impurities and increase the methane content of biogas to over 95%, meeting stringent gas grid standards. This opens up new possibilities for decentralized energy production and creates a valuable resource from what was once considered waste.
Industrial symbiosis: Kalundborg Eco-Industrial Park as a model for circular economy
Industrial symbiosis is a key concept in the circular economy, where waste or byproducts from one industry become valuable inputs for another. The Kalundborg Eco-Industrial Park in Denmark is a pioneering example of this approach in action. In this industrial ecosystem, companies exchange materials, energy, and water in a closed-loop system, significantly reducing waste and resource consumption.
For instance, excess steam from a power plant is used by a nearby pharmaceutical company, while gypsum produced as a byproduct of the power plant's desulfurization process is used by a wallboard manufacturer. This collaborative approach not only reduces environmental impact but also creates economic benefits for all participating companies. The success of Kalundborg has inspired similar eco-industrial parks worldwide, demonstrating the potential of industrial symbiosis to drive sustainability at scale.
Carbon capture and utilization (CCU) innovations
As the world grapples with the urgent need to reduce greenhouse gas emissions, Carbon Capture and Utilization (CCU) technologies are emerging as crucial tools in the fight against climate change. These innovations aim not only to remove carbon dioxide from the atmosphere but also to transform it into valuable products, creating economic incentives for carbon reduction.
Direct Air Capture (DAC) technologies: climeworks and carbon engineering approaches
Direct Air Capture (DAC) technologies are pushing the boundaries of carbon removal by extracting CO2 directly from the ambient air. Companies like Climeworks and Carbon Engineering are at the forefront of this field, developing large-scale DAC facilities that can remove thousands of tons of CO2 from the atmosphere annually.
Climeworks uses a modular approach with fans that draw air over a selective filter material that captures CO2. Once the filter is saturated, it's heated to release the concentrated CO2, which can then be stored underground or used in various applications. Carbon Engineering, on the other hand, employs a liquid-based system that chemically scrubs CO2 from the air, allowing for continuous operation and scalability.
Co2-to-fuels conversion: artificial photosynthesis and electrochemical reduction
The concept of converting captured CO2 into useful fuels is gaining momentum as a way to create a carbon-neutral fuel cycle. Artificial photosynthesis mimics the natural process used by plants to convert sunlight, water, and CO2 into energy. Researchers are developing catalysts and systems that can efficiently split water into hydrogen and oxygen, then combine the hydrogen with CO2 to produce hydrocarbons like methanol or ethanol.
Electrochemical reduction of CO2 is another promising approach. This process uses electricity to drive chemical reactions that convert CO2 into valuable chemical feedstocks or fuels. Recent advancements in electrode materials and system design have significantly improved the efficiency and selectivity of this process, bringing it closer to commercial viability. As renewable energy becomes more abundant and cheaper, these electrochemical processes could provide a sustainable pathway for producing carbon-neutral fuels and chemicals.
Biological carbon sequestration: engineered microalgae and enhanced weathering
Nature has been sequestering carbon for millions of years, and scientists are now looking to enhance these natural processes. Engineered microalgae strains are being developed to capture CO2 more efficiently than their natural counterparts. These supercharged algae can be cultivated in bioreactors or open ponds, converting CO2 into biomass that can be used for biofuels, food supplements, or other products.
Enhanced weathering is a technique that accelerates the natural process by which rocks absorb CO2 from the atmosphere. By crushing certain types of rocks and spreading them over large areas of land or sea, researchers aim to significantly increase the rate of CO2 absorption. This approach not only removes carbon from the atmosphere but also can improve soil quality and potentially increase crop yields, offering multiple benefits beyond carbon sequestration.